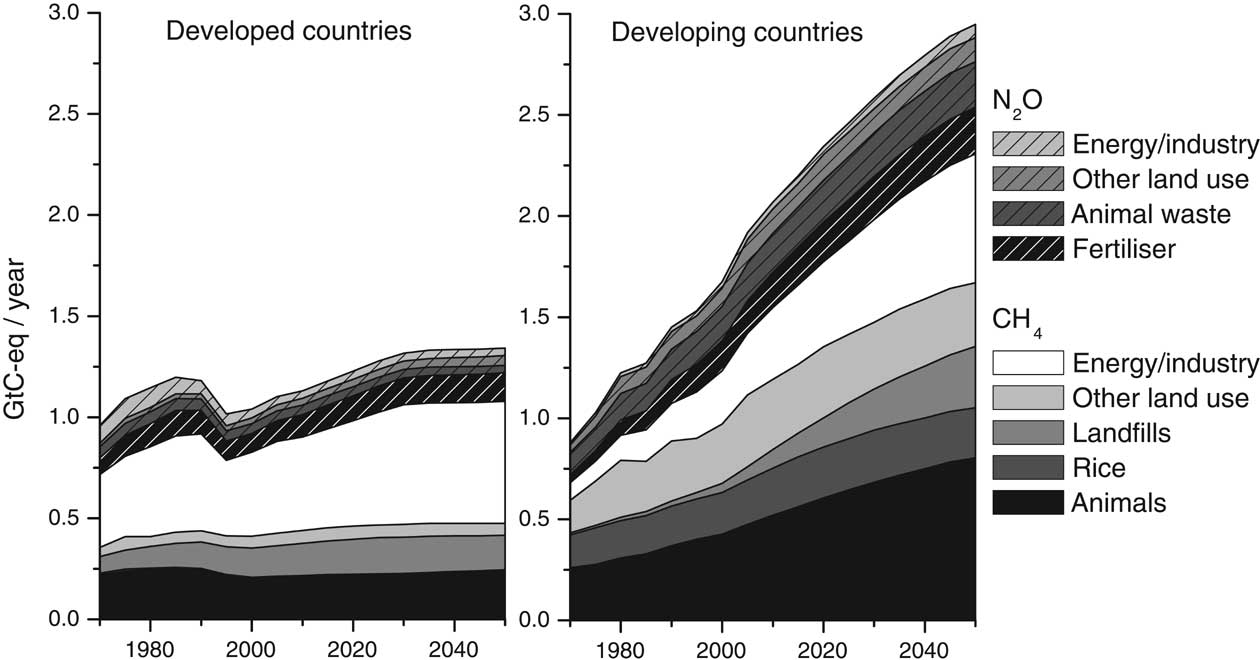
Figure 4-26. Estimated historical and projected N2O and CH4 emissions from 1970-2050. Source: Van Vuuren et al., 2007.
house gases (GHGs) (Figure 4-26). This particularly holds for methane (CH4) and nitrous oxide (N2O), both with higher global warming potential than CO2. Methane emissions are primarily caused by livestock production and flooded rice fields, while N2O emissions are related to the use of organic and inorganic N fertilizers. Finally, CO2 emissions are also caused by land use changes and agricultural practices.
Several studies have found that reducing non-CO2 greenhouse gases from agriculture and CO2 emissions from land use change can effectively reduce emissions. These mul-tigas emissions reduction scenarios are able to meet climate targets at substantially lower costs for the same targets as was found in the EMF-21 study (IPCC, 2007c; Lucas et al., 2007). A variety of options exists for mitigation of GHG emissions in agriculture (Table 4-13). Effective options are improved crop and grazing land management, restoration of drained organic (peat) soils and restoration of degraded lands (IPCC, 2007c). Lower, but still significant mitigation is possible with improved water and rice management, conservation plots, land use change and agroforestry. The relevant measures depend highly on the carbon price, i.e., the market price for reducing GHG emissions. At low carbon prices, profitable strategies are minor changes in present production systems, such as changes in tillage, fertilizer application, livestock diet formulation and manure management. Higher prices could allow the use of costly animal feed-based mitigation options, or lead to changes in land use. Effective options however, depend in general on local conditions, including climate, agricultural practices and socioeconomic circumstances; there is no universally applicable list of effective options (IPCC, 2007c).
4.4.4.5 Consequences forAKST
Based on the discussion above, challenges for AKST in the field of agriculture consist of:
• Adaptation. As climate change is likely to result in at least 2°C warming by the end of the century, and possibly >6°C, agricultural systems need to adapt to climate change. This will be even more important in developing countries than in developed countries. Effective adaptation should focus on extremes as well as changes in means. AKST will need to create less vulnerable system and AKST actors will need to provide information on options for adaptation. |
|
• Mitigation. Agriculture is also a major source of emissions. Although some technologies already exist to reduce CH4 and N2O emissions from agriculture, further progress is required to reduce emissions beyond 2020.
4.4.5 Energy
4.4.5.1 Trends in world energy use
There are very important relationships between energy and agriculture. The industrial revolution led to improved access to energy services based on fossil energy (e.g., Smil, 1991) and allowed for higher production levels per unit of land or labor in the agriculture sector. In turn, this allowed for a dramatic increase in the global population, a (related) decrease in arable land per capita, and a movement of the work force away from agricultural production.
Global energy use during the last century increased by about 2.5% annually, with a clear transition in consumption of primary sources from coal to oil to natural gas (Figure 4-27). The large majority of current scenarios project a continuation of these trends. Global energy use continues to grow; in the first decades growth will primarily be based on fossil fuel consumption. Primary energy consumption projections can be found in the IPCC-SRES scenarios, World Energy Outlook (IEA, 2002; IEA, 2004) United States Department of Energy (US DoE, 2004) and the OECD environmental outlook (OECD, 2006a). The differences between the different scenarios (Figure 4-28) can be explained in terms of underlying economic growth assumptions and assumed emphasis on dematerialization. In nearly every scenario, the largest contribution to global energy increase comes from developing countries. The scenarios also share the projection that in 2030 the majority of global energy use needs come from fossil fuels. Nevertheless, clear differences in the energy mix may occur. The most important determinants are the stringency of future climate policy, differences in technology expectation, and assumed societal preferences. Studies also indicate that global energy consumption could increase by 25-100% in the next 30 years, a huge challenge to production. In the longer term, the energy mix may diversify in many different ways, ranging from almost total coal use (e.g., IPCC's A2 scenario) versus nearly total renewable energy (e.g., under stringent climate policy scenarios). The growing awareness of both the "global warming" and "peak oil" issues is finally forcing decision makers and the general public to put energy high on policy agendas.
4.4.5.2 The relationship between energy use and agriculture
The food and energy systems have historically interacted in several ways (e.g., Pimentel and Pimentel, 1979; Pimentel, 1980; Stanhill, 1984; Leach et al., 1986; Smil, 1987, 1991; Stout, 1991, 1992). As indicated above, a dominant trend during the last century was increasing energy use leading to continuous increasing productivity of agricultural land. The implication of this trend can be seen when comparing the performance of industrialized and developing countries, in the relationship between energy inputs and yields (Giampietro, 2002). Agricultural production represents only a small part of global energy consumption. However, energy consumption not only occurs in the production stage. In the EU, the food supply chain used nearly 4 EJ of primary en- |